The Nanotechnology Gold Rush
Mathias Brust
Department of Chemistry, University of Liverpool, Liverpool L2 4TE, United Kingdom
Computers have shaped our modern world more than any other invention. They became a realistic possibility only through the advent of microtechnology, which enabled the cheap mass production of integrated electronic circuits with previously unimaginable data processing power. Since computation speed and information density depend crucially on the level of integration and the feature size of the circuitry, further miniaturisation has been the mantra of the industry from its beginnings, naturally leading to the concept of nanotechnology. Extrapolating from the opportunities afforded by microtechnology, extraordinarily high expectations have been associated with nanoscale manufacturing, and vast sums of money have been invested in it over the past three decades. In this lecture, I will examine from the point of view of a colloid chemist, why these expectations have not been fulfilled, and which alternative opportunities have been created instead, notably in the life sciences and in medicine. In this context, I will discuss our research on the use of gold nanoparticles for both analytical/diagnostic and therapeutic purposes. Photoacoustic methods of tracking gold nanorod-labelled stem cells within a murine model, and photodynamic approaches to the generation of singlet oxygen as a cytotoxin to kill cancer cells will be presented.
While microscale manufacture is firmly based on top-down engineering principles and homogeneous and stable materials, nothing can be envisaged on the nanoscale without considering the grainyness of matter along with the dynamics and instability inherent to assemblies of such small size. These are typical properties found in biological systems, which are also organised on the nanoscale but, in addition, depend on the ability to self-repair and on an existence far from chemical equilibrium. Unlike manufactured nano-systems, they are indeed processes, and not objects. Again, using gold-based assemblies as an example, I will present and discuss approaches to making artificial nanostructures more process-like and conceptually more similar to biological phenomena. In particular, I will present gold nanoparticles that act as ion transporters across membranes and can maintain ion fluxes between emulsion droplets that are not in chemical equilibrium with each other.
Classical Nucleation Theory and Electrodeposition
W. Schwarzacher
H. H. Wills Physics Laboratory, University of Bristol, Tyndall Avenue, Bristol BS8 1TL UK
w.schwarzacher@bristol.ac.uk
In this talk I shall briefly cover the basics of classical nucleation theory using the nucleation of ice from liquid water as an example. I shall discuss what makes an effective heterogeneous nucleating agent for ice and why this is an important scientific problem. I shall then present some of our recent work using bio-nanoparticles as model iced nucleating agents. I shall finish by presenting an example of classical nucleation theory applied to electrodeposition as a warning that not every article published in a high impact factor journal should be believed.
Porous hypercrosslinked polymer-TiO2-graphene photocatalysts for visible-light-driven CO2 conversion
S. L. Wang1†, M. Xu1†, T. Y. Peng2, C. X. Zhang1, T. Li1, I. Hussain3, J. Y. Wang1★ and B. E. Tan1★
1Huazhong University of Science and Technology, Luoyu Road No. 1037, Wuhan, 430074, China.
2 Wuhan University, Bayi Road No. 299, Wuhan, 430072, China.
3Lahore University of Management Sciences (LUMS), Lahore, Pakistan.
*B. E. Tan, Tel: +086-027-87558172, bien.tan@mail.hust.edu.cn.
Significant efforts have been devoted to develop efficient visible-light-driven photocatalysts for the conversion of CO2 to chemical fuels1,2. The photocatalytic efficiency for this transformation largely depends on CO2 adsorption and diffusion. However, the CO2 adsorption on the surface of photocatalysts is generally low due to their low specific surface area and the lack of matched pores3-5. To overcome this limitation, a well-defined porous hypercrosslinked polymer-TiO2-graphene (HCP-TiO2-FG) sandwiched structure is reported with relatively high surface area i.e., 988 m2 g-1 and CO2 uptake capacity i.e., 12.87 wt% (Fig.1). Meanwhile, the HCPs obtained by this strategy are comprised of ultrathin layers with a thickness of 3~8 nm wrapping around TiO2 crystals, which facilitates the diffusion of CO2 molecules from the adsorptive sites on HCPs layers to the catalytic sites on TiO2 photocatalysts (Fig.2). Due to the improved CO2 adsorption ability and shortened diffusion length, such well-defined HCP-TiO2-FG sandwich photocatalyst is expected to enhance the reactivity of CO2 molecules, which will facilitate the CO2 conversion especially for CH4 production (Fig.3).
This sandwiched
structure shows high photocatalytic performance especially for CH4
production, i.e., 27.62 μmol g-1 h-1, under mild reaction
conditions without the use of sacrificial reagents or precious metal
co-catalysts. The enhanced CO2 reactivity can be ascribed to their improved
CO2 adsorption and diffusion, visible-light absorption, and photo-generated
charge separation efficiency. It can be concluded that the in-situ knitting of
porous HCP-TiO2-FG sandwich structure are very effective to
dramatically enhance visible-light-driven CO2 conversion efficiency,
which could be mainly ascribed to the well-defined sandwich structure from the
following two aspects: (1) high CO2 uptake ability and short
distance between the adsorbent and the catalyst facilitating the CO2
adsorption and diffusion; (2) broad light absorption of HCPs layers and fast
charge mobility of graphene improving the visible-light absorption and charge
separation efficiency (Fig.3). This strategy provides new insights into the
design and synthesis of well-defined porous photocatalysts for CO2 uptake
and conversion, and highlights the importance of microporous organic polymers
in combination with photocatalysts for solar energy conversion.
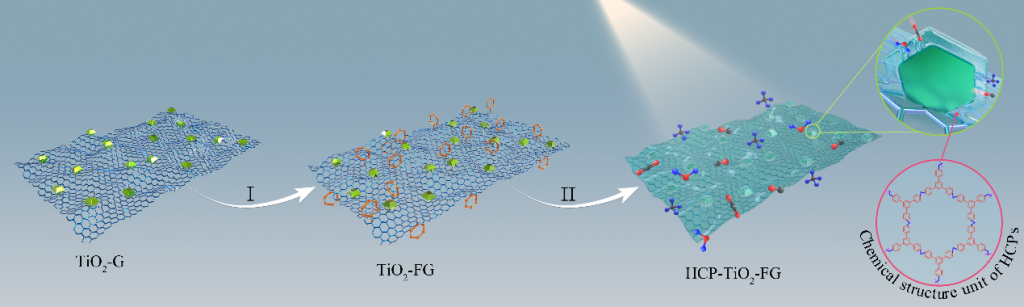
Figure 1. Construction of a well-defined porous HCP-TiO2-FG sandwich structure.
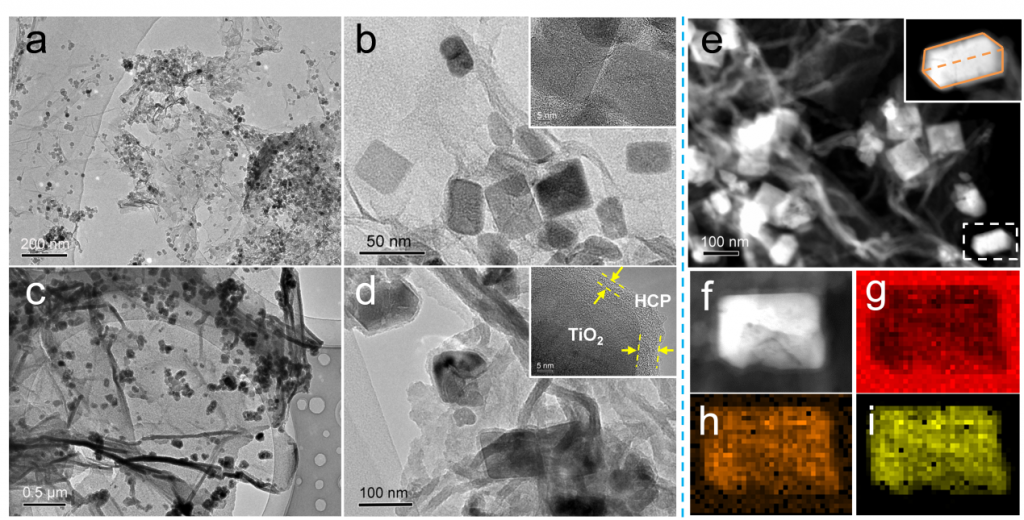
Figure 2. Morphology and elemental mapping of various photocatalysts. TEM images of TiO2-G (a, b) and HCP-TiO2-FG (c, d) at different magnification. The insets in b and d are the corresponding HR-TEM images. STEM images of HCP-TiO2-FG (e, f). EDX mapping images of C (g), O (h), and Ti (i) for HCP-TiO2-FG.
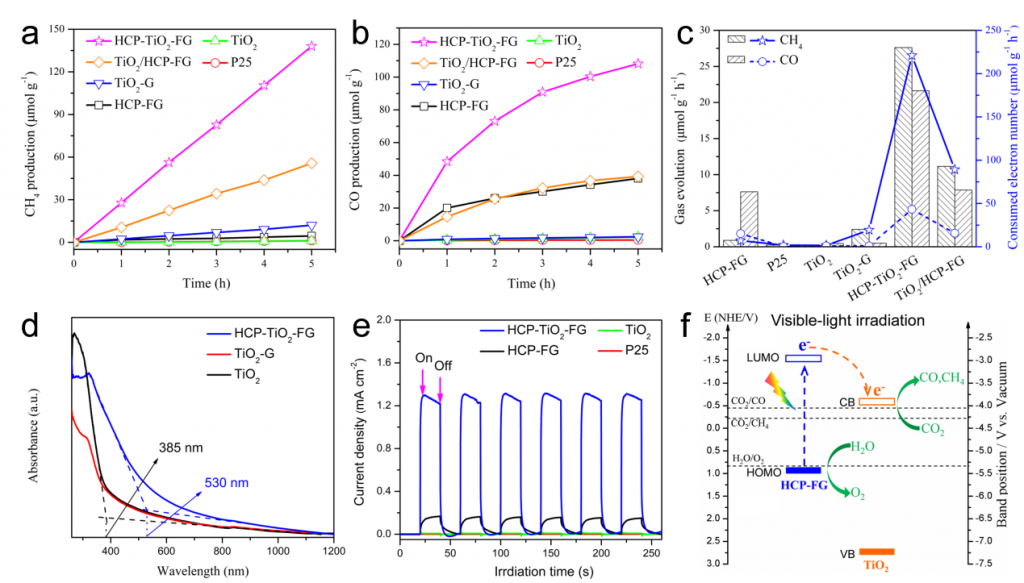
Figure 3. The photocatalytic performance of CO2 reduction, optical and photoelectrical properties, and mechanism of charge transfer pathway. Time-dependent production of CH4 (a) and CO (b) in photocatalytic CO2 reduction with different catalysts under visible-light (λ≥420 nm). (c) Average efficiency of photocatalytic CO2 conversion with different catalysts during 5 h of visible-light (λ≥420 nm) irradiation. (d) UV-Vis absorption spectra of TiO2, TiO2-G, and HCP-TiO2-FG catalysts. (e) Amperometric I−t curves of samples under visible-light (λ≥420 nm) irradiation. (f) Proposed mechanism of charge separation and transfer within the HCP-TiO2-FG sandwich photocatalyst under visible-light (λ ≥420 nm) irradiation.
References
[1] K. Li, B. Peng & T, Peng. ACS Catal. 6 (2016), pp. 7485-7527.
[2] X. Qiao. et al. J. Am. Chem. Soc. 139 (2017), pp. 3934-3937.
[3] T. Nakajima. et al. J. Am. Chem. Soc. 138 (2016), pp. 13818-13821.
[4] H. Rao. L. C. Schmidt, J. Bonin, & M. Robert. Nature 548(2017), pp. 74-77.
[5] N. Elgrishi, M. B. Chambers, X. Wang & M. Fontecave. Chem. Soc. Rev. 46 (2017), pp. 761-796.
Sequence-Specific “Peptoids” for Bionano Science and Engineering
K. H. Aaron LAU
Senior Lecturer (Associate Professor), Department of Pure and Applied Chemistry, University of Strathclyde, Glasgow, Scotland, United Kingdom
Analogous to biopolymers such as peptides and DNA, biomimetic “peptoids” are synthetic polymers that exhibit complex self-assembly properties and bioactivity encoded by the specific order in which simple monomer residues are arranged in linear sequences. In contrast to biopolymers, peptoids are resistant to enzyme degradation and have simplified intermolecular interactions. This talk highlights our recent efforts in “engineering” the sequence of peptoid solid-phase synthesized peptoids for applications beyond their convention use in combinatorial drug discovery. We demonstrate a series of novel properties, including the shortest peptoid motifs found to self-assembly of into nanospheres and nanofibrils, “stealth” surface-grafted peptoid brushes that resist biofouling, as well as the modification of known sequences to retain antimicrobial activity for biomedical surface applications. Our preliminary results also show that self-assembled peptoid nanosheets can influence protein expression of stem cells under culture.
The Effect of Confinement on the Crystallization of Soft-Materials
Hatice Duran
Associate Professor, TOBB University of Economics and Technology, Department of Materials Science & Nanotechnology Engineering, Sogutozu Cad. 43 06560, Ankara, TURKEY
Nanoporous hard templates provide a two-dimensionally confined space in which self-organization processes such as crystallization, protein secondary structure formation, and phase separation can be fundamentally different from those obtained in thin films and in the bulk. A particular advantage of hard templates is that they provide a range of parameter space (pore diameter, curvature, nature of pore walls) that can induce or manipulate nucleation and crystal growth. Nowadays, a broad range of soft materials can be formed into nanotubes by means of nanoporous hard templates containing arrays of self-ordered cylindrical nanopores. Understanding the crystallization, thermodynamics and dynamics of soft materials under confinement allow for their rational design as functional devices with tunable mechanical strength, processability, electronic and optical properties. The principal focus of this lecture is finding the basic underlying principles that give rise to nucleation and crystal growth in a range of soft materials (crystallizable polymers, amphiphilic molecules, liquid crystals, low-molecular weight liquids and biopolymers) under hard-confinement.
We employed self-ordered nanoporous aluminum oxide (AAO) made by the electrochemical anodization of aluminum substrates as the inorganic model matrix that provides the required uniformity in diameter/length, thermal stability and resistance to organic solvents. In addition, the parallel arrangement of the pores in AAO allows for studies of anisotropic properties of crystal textures and even crystal dynamics parallel and perpendicular to the pore walls. In recent years it was shown that a size limitation in the nanometer scale can affect the energetics involved in crystal nucleation, growth and orientation. In this talk, we will mention the controlled crystal orientation in semi-crystalline polymers, liquid-crystals and even water molecules in nanoconfinement.
Bulk crystallization of polymers proceeds usually via heterogeneous nucleation and gives rise to structure over multiple length scales: from the crystalline unit cell (1-2 nm), to alternating crystalline/amorphous lamellae (50-100 nm) and to spherulitic superstructures (>500 nm and up to cm). This is the dominant nucleation mechanism in bulk polymers. Important studies of polymer crystallization confined to droplets and within the spherical nanodomains of block copolymers emphasized the interplay between heterogeneous and homogeneous nucleation and explored the kinetics of homogeneous nucleation observed at high supercooling. Some authors have even reported only homogeneous nucleation of polymers confined to AAO nanopores. This finding is surprising and leads to several questions about the type of nucleation under hard confinement typical for AAO: Is the crystallization process always homogeneous within the small AAO nanopores? Do surface effects always dominate? And even more, can hard confinement completely suppress polymer crystallization and if so what is the required size? Providing answers to these questions is of technological relevance for the understanding of nanocomposites containing semi-crystalline polymers. We will try to explore these issues both by comparing the recent studies and by presenting research activities of our group.
Nanotechnology: key to synthetic fuels for a sustainable energy transition
Asif Ansar
Head of High Temperature Electrochemical Energy Systems & Processes, German Aerospace Center (DLR), Stuttgart, Germany.
Worldwide energy consumption in the last 50 years has grown from around 40K TWh to more than 150K TWh. At the same time, the unprecedented increase in the emissions of greenhouse gases and other pollutants, resulting in smog, has hard-pressed the society to look for cleaner energy and mobility scenarios. Society will continue to need more energy while demanding reduction in emissions and if it is to succeed in this formidable challenge it must strike the right balance between cost, efficiency, scalability, and environmental impact. To attain these ambitions, the energy supply scenario will remain a complex mix with increasing share of intermittent renewable energy. This intermittency in power generation due to renewable power and in power demand mainly due to emerging electro-mobility will put stringent pressure on our energy supply-demand patterns.
With this background, the lecture will outline the keys to a secure and sustainable future energy supply: energy storage, grid balancing and sector coupling. To implement these, technologies for power-to-chemicals including synthetic “E”-fuels and electrolytic hydrogen as the building blocks will be needed. The lecture will explain how nanotechnology is one of the essential enablers of the technologies. The challenges and the current progress in nanotechnology for water electrolysis for green hydrogen and co-electrolysis for the production of synthetic hydrocarbon will be detailed. As the market adaptation of these technologies is driven by the cost of the produced fuel, the technological development towards lower cost will be discussed. In the end, the prototype plants of German Aerospace Center for water and co-electrolysis coupled with Fischer-Tropsch-Synthesis will be presented.
BioSurfactant Stabilised Nanomaterials – Antimicrobial, Cytotoxic Activity
Mohammed Yaseen1*, Kathleen Kuremyr1, Majed Bawazeer1, Andrew Hursthouse1, Trevour Ludlam2, Robert Wilson2
1School of Computing, Eng. and Physical Sciences, University of the West of Scotland UK
2Creative Chemicals Ltd, UK
Email: mohammed.yaseen@uws.ac.uk
The global emergence and spread of antibiotic and general drug resistance inherently has led to problems in the effective disease treatment and hygiene within built environments. Thus novel strategies to treat and prevent infections as well as control hygiene are urgently required. Apart from selective antimicrobials including biosurfactants, the use of metal ions such as silver and copper as well as chlorine and peroxide is well known.
In this regard, we have synthesised silver nanoparticles (NPs) by simple reduction of silver salts to produce silver spherical and plates coated with zwitterionic, ionic and non-ionic amphilic molecules. The surface charge of NPs, their size and shape were determined using dynamic light scattering and Electron Microscopy. Antimicrobial testing against E. coli, P. Aeruginosa and S. Aureus showed signicant > 90% antimicrobial activityfor NPs coated with zwitterionic and ionic surfactants compared to low activity of NPs and surfactant alone. Further cytotoxic testing against against Hela, MCF-10A, Hep-G2 and MCF-7 cells showed comparable activity to that of Tamoxifen for NPs coated with lower concentration of surfactants. The results show promising antibacterial and cytotoxic activity of synergistic NPs – biosurfactants, which on their own would require either higher concentrations leading to resistance or have little activity. Further work on influence of shape and the mechanism of activity is being carried out now.